Concurrent Supplementation of Arginine, Vitamin E, and Vitamin C Improve Cardiopulmonary Performance in Broilers Chickens
Concurrent supplementation of arginine and vitamins C and E significantly improved the pulmonary vasodilation of broiler chickens kept at low temperature, according to research from DSM Nutritional Products. Further research is warranted to elucidate these mechanisms, say the researchers, but the dietary treatment appears to reduce ascites.Abstract
Two experiments were conducted to evaluate the effects of arginine, vitamin E (VE), and vitamin C (VC) on cardiopulmonary performance and ascites parameters of broilers reared under a cold environmental temperature. One-day-old male broilers were fed a basal corn-soybean meal diet (control, 1.2 per cent arginine and 40 IU of VE), or the basal diet supplemented with 1 per cent arginine and either 200 IU vitamin E (AE), 500 mg of vitamin C (AC), or a combination of VE and VC at the same amounts (AEC) per kilogram of feed. Pulmonary arterial pressure (PAP) and mean arterial pressure were recorded in clinically healthy, anesthetized birds (28 to 42 d old) before and after an epinephrine (Epi) challenge (0.5 mg/kg of BW, i.v.), an aminoguanidine hemisulfate challenge (100 mg/kg of BW, i.v.), and an N-nitro-l-arginine methyl ester challenge (50 mg/kg of BW, i.v.) at 20-min intervals. Data were analyzed by repeated measures ANOVA, and the Student Newman-Keuls test was used to separate means within groups. The PAP increased 30 s after the Epi challenge in all birds, but the peak PAP was lower in the AEC group than in all the other groups, whereas birds in the AE and AC groups had lower PAP peaks than did the control group. After 120 s of challenge, the PAP was lower in AEC birds compared with the other birds. The PAP returned to pre-Epi amounts within 300 s in all groups. The PAP was increased (P<0.05) within 60 seconds after the aminoguanidine hemisulfate and N-nitro-l-arginine methyl ester challenges in all groups, but no differences were found among groups. The mean arterial pressure responses did not differ among groups. Plasma NO was greater in the AEC group than in all the other groups before and after the Epi challenge. These results showed that Epi elicited lower amplitude PAP and less prolonged increases in PAP in birds from the AEC group, and this may have been related to the increased vasodilation attributable to NO production. The AEC may have had complementary effects against oxidative stress, protecting the endothelium and preserving NO function.
Introduction
Pulmonary hypertension syndrome (PHS, ascites) is a common metabolic disorder of modern, fast-growing strains of broilers. Ascites mortality peaks close to market age when the birds are five to six weeks old, which represents a significant loss to the industry, given the cost of production up to this point (Maxwell and Robertson, 1998). Ascites can be initiated by an increase in metabolic rate, which implies a greater oxygen demand (Scheele et al., 1991; Buys et al., 1999; Wideman and Tackett, 2000). In a gas exchange system working close to its physiological limit, this elevation in metabolic demand can lead to cellular hypoxia (Scheele et al., 1991). To avoid cellular hypoxia, an increased cardiac output must be propelled through the noncompliant pulmonary vasculature, which requires increases in pulmonary arterial pressure (PAP) and right ventricle (RV) work (Wideman, 1988). Sustained increases in PAP cause hypertrophy and subsequent dilation of the RV (Peacock et al., 1990; Wideman, 1999).
By reducing the pulmonary vascular resistance, it is possible to reduce the PAP needed to propel the cardiac output required to match the metabolic demands of the broilers (Wideman et al., 1995) and delay the pathophysiological progression leading to ascites. Nitric oxide is an important endogenous vasodilator produced by endothelial cells through an oxygen-dependent five-electron oxidation of a terminal guanidine nitrogen of l-arginine (ARG; McQueston et al., 1993; Palmer et al., 1998). l-Arginine is an essential amino acid in birds (Tamir and Ratner, 1963), and it has been reported that ARG amounts supporting the maximal growth rate are not adequate for maximal NO production (Taylor et al., 1992; Dietert and Austic, 1994).
Supplemental ARG reduced the incidence of ascites in broilers exposed to a cool temperature (Wideman et al., 1995; Tan et al., 2005), improved pulmonary vasodilation (Wideman et al., 1996; Lorenzoni and Ruiz-Feria, 2006), and facilitated vasorelaxation in fowl (Martinez- Lemus et al., 1999). However, the effects of ARG on ascites incidence have not been consistent (Ruiz-Feria et al., 2001).
Oxidative stress is also involved in the aetiology of PHS (Peacock et al., 1990; Maxwell et al., 1992; Enkvetchakul et al., 1993; Bottje and Wideman, 1995; Grobe et al., 2006). A sustained high PAP is associated with hypoxia, endothelial damage, and reactive oxygen species production. Chronic hypoxia increases ROS concentrations and impairs endothelial NO-dependent relaxation in mice pulmonary arteries (Fresquet et al., 2006). The superoxide anion causes a loss of NO bioavailability by shortening its half-life (Jung et al., 2003), thereby reducing the potential for endothelial vasodilation (Lopez-Lopez et al., 2001). Furthermore, the reaction of superoxide anion with NO leads to production of peroxynitrite, a potent oxidant agent responsible for direct tissue damage (Beckman et al., 1990; Szabo, 1996).
Ascitic broilers have been reported to have low concentrations of vitamin E (VE; Bottje et al., 1995) and ascorbic acid (Enkvetchakul et al., 1993) in the lungs and liver. Vitamin E supplementation reduced the incidence of ascites when administered as a subcutaneous implant, but not when provided as a dietary supplement (Bottje et al., 1995, 1997). We previously reported that VE at 400 IU/kg of feed, when supplemented in combination with ARG, was associated with less effective pulmonary vasodilation after an epinephrine (Epi) challenge, compared with birds receiving only ARG (Lorenzoni and Ruiz-Feria, 2006). In addition, birds receiving ARG and large amounts of VE had greater concentrations of TBA-reactive substances than birds fed normal diets, suggesting that large amounts of VE increased oxidative stress. Vitamin E reacts with organic peroxides, interrupting the chain reaction of lipid peroxidation involving the formation of tocopheroxy radicals, which can act as prooxidative agents and initiate oxidative processes (Schneider, 2005).
Ascorbate has been shown to prevent the prooxidant activity of a-tocopherol by reducing the a-tocopheroxyl radical to a-tocopherol, and then acting as a coantioxidant (Carr et al., 2000). Through this mechanism, vitamin C (VC) supplementation has been reported to increase or restore plasma and tissue VE (Liu and Lee, 1998; Keller et al., 2004). Furthermore, ascorbate has been reported to have beneficial effects on endothelium-dependent vasodilation by decreasing the concentrations of superoxide radicals, which inactivate NO (Dudgeon et al., 1998). Vitamin C, when supplemented in feed at 500 mg/kg, reduced the incidence of PHS in broilers (Ladmakhi et al., 1997; Xiang et al., 2002). However, diets containing flax oil, VE and VC increased the incidence of ascites in broilers (Walton et al., 2001).
The combined effects of ARG, VE and VC on cardiopulmonary performance and ascites parameters have not been studied. The authors hypothesized that ARG and antioxidant vitamins may have complementary effects on cardiovascular performance, with ARG providing extra substrate for NO production, and vitamins E and C providing protection against oxidative stress. In this study, we evaluated the effects of ARG and VE or VC, or a combination of ARG, VE and VC on cardiopulmonary responses after an acute Epi challenge and subsequent intravenous injections of aminoguanidine hemisulfate (AG) and N-nitro-l-arginine methyl ester (l-NAME) on plasma concentrations of NO, and on the hematocrit values of broiler chickens reared under cold exposure.
Materials and Methods
A total of 96 one-day-old male Cobb 500 broiler chicks were used in each of two experiments. Chicks were wing-banded and reared in light-, temperature-, and ventilation-controlled environmental chambers. All broilers were brooded at the recommended brooding temperatures until 16 days of age. Thereafter, all broilers were subjected to a cool temperature (16°C) until six weeks of age to amplify the incidence of PHS. The data obtained from the two experiments were pooled before statistical analysis.
All chicks were fed a basal corn-soybean meal diet to meet or exceed the NRC (1994) requirements, including 22.5 per cent CP and 3,150 kcal/kg of ME (1 to 21 d), or 19 per cent CP and 3,250 kcal/kg of ME (22 to 42 d). The basal diet included ARG at 1.2 per cent (wt/wt) and VE at 40 IU/kg of feed for both growing periods; the details of the diet composition, along with micronutrients, are described elsewhere (Baurhoo et al., 2007). Birds were divided into 4 groups: a control group (CTL, basal diet), a high-ARG and high-VE group [AE; basal diet plus 1 per cent supplemental l-arginine monohydrochloride (Sigma, Oakville, Ontario, Canada), and 200 IU of dl- a-tocopheryl acetate (Rovimix E 50 SD, DSM nutritional products, Ayr, Ontario, Canada)], a high-ARG and VC group (AC>; basal diet plus one per cent ARG and 500 mg/kg of ascorbic acid; Sigma), and a high-ARG, high- VE, and VC group (AEC basal diet plus one per cent ARG, 200 IU of a-tocopherol acetate, and 500 mg/kg of VC). The diets were not pelleted and were mixed in small batches to prevent inactivation of the ascorbic acid.
Surgery
From days 28 to 42, clinically healthy birds were selected for the evaluation of cardiopulmonary performance (n=7 and n=16 per group for experiments 1 and 2, respectively). Birds were anaesthetized to a surgical plane with allobarbital (5,5-diallyl-barbituric acid, Dial mixture, Sigma, St. Louis, MO; 50 mg/kg of BW i.m.) and lidocaine hydrochloride (Xylocaine, Astra- Zeneca Canada Inc., Mississauga, Ontario, Canada; 2 per cent s.c.) as a supplemental local anaesthetic at the incision sites. Birds were fastened in dorsal recumbency, and the wings and legs were extended on a heated surgical board regulated to maintain a surface temperature of 30°C and a 20° head-up angle. The left brachial artery was isolated and cannulated with 30 cm of heparinised polyethylene tubing (PE-50, Becton Dickinson Canada Inc., Oakville, Ontario, Canada). The left brachial vein was cannulated by using 30 cm of heparinized Silastic tubing (0.012 i.d. × 0.025 o.d., VWR International, Mississauga, Ontario, Canada) and the proximal end was advanced through the vein and RV until it reached the pulmonary artery. Both distal ends of the PE-50 polyethylene tubing and the Silastic tubing were attached to blood pressure transducers interfaced with a Transbridge preamplifier to a Biopac MP100 data acquisition system using Acknowledge software (Biopac Systems Inc., Goleta, CA) for the continuous measurement of PAP (mm Hg), mean systemic arterial pressure (MAP, mm Hg), and heart rate (HR, beats/min).
Experimental Protocols
Once birds were cannulated, they were allowed to stabilize for 10 minutes. During this period, representative PAP and MAP readings were taken at 300 and 60 seconds before an Epi [4-(1-hydroxy-2-[methylamino]ethyl)-1,2- benzenediol hydrochloride, Sigma, St. Louis, MO] challenge (0.5 mg/kg of BW, i.v.), to obtain basal values. Epinephrine exerts a strong vasonstrictive effect (Smith et al., 2000), increasing MAP and PAP. Evaluation of the vasodilation capacity was estimated by measuring the increment of the PAP after each challenge and the time birds within each dietary treatment took to return to the basal measurement. The PAP, MAP, and HR responses were measured at 30, 60, 120, 300, 600, 720 and 1,200 seconds after the Epi challenge. At 1,200 seconds after the Epi challenge, birds were injected with AG (100 mg/kg of BW, i.v.), and the PAP, MAP, and HR were recorded at the same time intervals (30, 60, 120, 300, 600, 720 and 1,200 seconds after the AG challenge). A third challenge with l-NAME (50 mg/kg of BW, i.v.) was conducted 1,200 s after the AG challenge, and the same parameters were recorded at the interval times described previously.
The Biopac MP100 system monitored two primary channels, including MAP and PAP. Values for these parameters were averaged electronically during 10-s recordings at the above-mentioned representative sampling times. The protocol used for data averaging was previously demonstrated to compensate accurately for the influences of pulse pressure and respiratory cycles on PAP and MAP (Wideman et al., 1996). Heart rate was obtained by counting systolic peaks over time in the PAP recording coincident with each sampling time interval. At the end of the experiment, birds were humanely killed and the heart was dissected to determine the RV to total ventricle ratio (RV:TV) as an indicator of PHS (Burton et al., 1968; Cueva et al., 1974). All animal procedures were approved by the McGill University animal care committee.
Determination of NO in blood
Blood samples (2 mL) for NO determination were collected in Vacutainer (Becton Dickinson Canada Inc.) tubes containing EDTA at five minutes before the Epi challenge and at 20 minutes after the l-NAME challenge. The plasma was separated by centrifugation and stored at -20°C until analysis. Plasma NO concentration was determined by using a Nitrate/Nitrite Colorimetric Assay Kit (Cayman Chemical, Ann Arbor MI). This test is based on a two-step process that converts nitrate to nitrite by using nitrate reductase, followed by the addition of Griess reagents, which convert nitrite into a deep purple azo compound. Analyses were carried out in 96-well microtiter plates, and absorbance of the azo chromophore was measured at 540 nm.
Hematocrit, RV:TV Ratio and PHS Mortality
Hematocrit values were recorded from weeks 3 to 6. Blood samples were collected from the wing vein into blood capillary tubes from randomly selected broilers (n=8 to nine per group for experiment 1, and n=18 for experiment 2) and centrifuged at 9,000 × g for three minutes. Birds that died during the experimental period were examined for lesions of heart failure and ascitic fluid in the abdominal cavity. The heart was removed, the RV was carefully cut from the left ventricle, and the RV:TV ratio was calculated (Burton et al., 1968; Cueva et al., 1974). At the end of six weeks, all birds were humanely killed and RV:TV ratios were calculated.
Statistical analysis
Body weight, RV:TV ratio, hematocrit, plasma NO and values within sampling points for PAP, MAP, and HR were analyzed by one-way ANOVA, and means were separated by the Student-Newman-Keuls method in both experiments. Plasma concentrations of NO before and after challenge within treatment group were compared by using a paired t-test, and differences were declared at P<0.05. The PAP, MAP, and HR were analyzed within treatment group over time by using the repeated measures ANOVA of SigmaStat (Jandel Scientific, 1994), and means were separated by the Student- Newman-Keuls method. Sampling times at -300 and -60 seconds were used as basal values for the Epi challenge. The sampling time of 1,200 seconds after the Epi challenge was considered the basal measurement for the AG challenge, and the sampling time of 2,400 seconds was considered the basal measurement for the l-NAME challenge. Differences were declared when the PAP, MAP, and HR during the challenges were statistically different (P<0.05) from their respective baseline values. Data for PAP, MAP, HR, and plasma NO were highly consistent in both experiments and were pooled before analysis. A value of P<0.05 was considered statistically significant.
Results and Discussion
The pulmonary arterial relaxation was improved when ARG was supplemented above the NRC (1994) requirements, and this has been attributed to an increase in NO production (Wideman et al., 1996; Lorenzoni and Ruiz-Feria, 2006). In addition, antioxidants such as VE (Bottje et al., 1995) and VC (Xiang et al., 2002) have been shown to reduce PHS mortality. However, the effects of ARG, VE, or VC on cardiopulmonary function and ascites incidence have not been consistent when used alone (Ruiz-Feria et al., 2001; Lorenzoni and Ruiz-Feria, 2006; Walton et al., 2001). The authors hypothesised that ARG and antioxidant vitamins may have complementary effects on cardiopulmonary performance, with ARG providing extra substrate for NO production, and antioxidant vitamins providing protection against oxidative stress and increasing NO bioavailability.
The basal PAP (before challenge) was not different among clinically healthy broilers in the different treatment groups (Figure 1; time -300 and -60 seconds). There was a significant increase in PAP at 30 s after the Epi challenge within all treatment groups (P<0.05; time 30 s vs. basal PAP). The PAP after the Epi challenge remained greater, compared with the basal measurements, for up to 300 s in the CTL and AE groups, but for less than 300 s in the AC and AEC groups. Furthermore, when comparing the PAP among treatments at the 30-s time (the peak response in PAP), the PAP was lowest (P < 0.05) in the AEC group and greatest in the CTL group, whereas the AE and AC groups had an intermediate (lower than the CTL group, but greater than the AEC group) and comparable PAP. At the 60-, 120-, and 300-s times, the PAP of the CTL group remained greater than the PAP of the AEC group, but was not different from the PAP of the AE and AC groups. At 60 s after the Epi challenge, the PAP of the AEC group was lower than the PAP of the AC group, but was not different from the PAP of the AE group. At 120 s after the challenge, the PAP of the AEC group was lower than the PAP of both the AE and AC groups, but at 300 s after the Epi challenge, there were no differences among the PAP of the AE, AC, and AEC groups. After the 600-s recording, the PAP was not different among treatments.
Therefore, the birds in the AEC group showed the best pulmonary vasodilation response after an acute Epi challenge, as evidenced by the lower peak in PAP at 30 s, the lower PAP from 60 to 300 s, and the rapid return of the PAP to basal measurements, whereas the birds in the AE and AC groups had a better pulmonary vasodilation response than the CTL birds. These results suggest an additive effect of ARG, VE, and VC on the cardiopulmonary response. The extra plasma concentrations of ARG may become available for enzymatic formation of NO, whereas antioxidant vitamins may protect the bioavailability of NO and protect the endothelial integrity, and in this way, improve the vasodilatory capacity of the lungs. In that regard, our results also indicated that VE or VC, in combination with ARG, provided limited protection against oxidative stress, but when both antioxidant vitamins were combined, the protective effect was enhanced. For instance, the superoxide anion (·O2 -) caused a loss of NO bioavailability by shortening its half-life, causing, among other effects, a decrease in endothelial vasodilation (Lopez-Lopez et al., 2001). Furthermore, the reaction of·O2 - with NO led to the production of peroxynitrite, a potent oxidant agent responsible for direct tissue damage by oxidation, peroxidation, and nitration of lipids, proteins, and DNA (Beckman et al., 1990; Szabo, 1996). The additive effects of VE and VC on antioxidant capacity have been reported in laying hens reared at high temperatures (Sahin et al., 2002) and in apolipoprotein E-deficient mice (Nespereira et al., 2003; Rodriguez-Gomez et al., 2005). It has been documented that VC ensures a-tocopherol regeneration from the a-tocopheroxy radical, thereby preventing VE prooxidant activity, acting as a “coantioxidant,” and inhibiting oxidation (Carr et al., 2000). We previously reported that VE supplementation (400 IU/kg of feed), along with ARG, increased TBA-reactive substances in the plasma of broilers reared in cold environments (Lorenzoni and Ruiz-Feria, 2006). Chickens can synthesize VC, but under intensive farming conditions and stressors such as rapid growth, heat, or cold, birds may be unable to synthesize adequate amounts of VC (Pardue and Thaxton, 1986). In addition, ARG may become a prooxidative agent under some circumstances; for instance, greater concentrations of NO production have been associated with the production of superoxide radicals (Hishikawa and Lüscher, 1997), and Ruiz-Feria et al. (2004) reported that high concentrations of ARG were associated with increased endothelial lesions in the aorta of birds, presumably because of increased oxidative stress. The results of these experiments strongly suggest that the combination of VE and VC had additive effects on improving cardiopulmonary performance and reducing pulmonary hypertension, and these may have been mediated by reductions in oxidative stress and an increased availability of NO, as discussed below.
Aminoguanidine is a specific inhibitor of the inducible form of nitric oxide synthase (NOS). After the AG challenge, the PAP values increased (P < 0.05) in all groups within 30 s, compared with basal values (time 1,200 s), and returned to the basal measurements after 120 s in all treatments, except in the AEC group, in which PAP returned to the basal measurement before 120 s. The peak of PAP was recorded at 60 s after the AG challenge in all treatments (time 1,260 s; Figure 1); however, the peak was greater in the CTL group than in the AEC group, whereas the AE and AC groups had an intermediate PAP peak, which was not different from the peak PAP of the CTL or AEC group. Wideman et al. (2006) reported that AG stabilized, but did not increase, the PAP during the period preceding microparticle injection; similarly, Bowen et al. (2006) reported that the PAP did not increase in either ascites-susceptible or ascites-resistant lines of broilers within 10 min after AG injection. On the other hand, Teshfam et al. (2006) reported that cold exposure increased endothelial NOS and inducible NOS (iNOS) gene expression, and Schroeder et al. (2000) observed that rats that developed hepatic and pulmonary hypertension presented a 9-fold increase in iNOS activity in the pulmonary endothelium. Therefore, the increase in PAP recorded after the AG challenge in these experiments may be the result of cold temperature exposure and birds developing PHS, further supporting the complementary effects of ARG, VE, and VC in reducing the deleterious effects of cold exposure on pulmonary vasodilation.
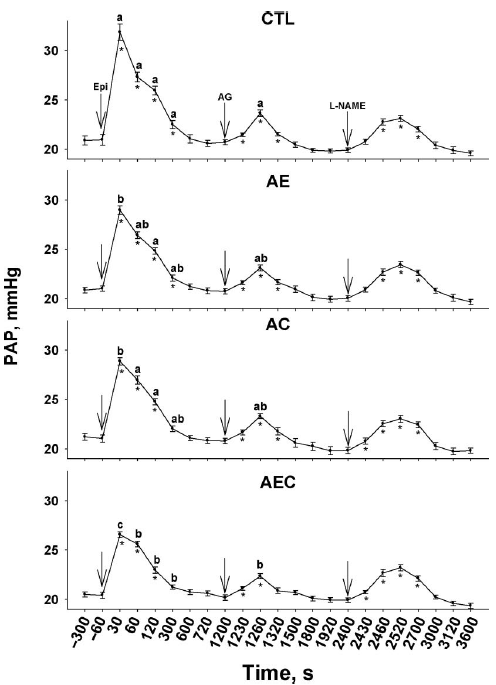
The PAP increased (P < 0.05) within 30 s after l- NAME injection in the AC and AEC groups, increased within 60 s in the CTL and AE groups, and remained elevated up to 300 s in all groups, but with no differences among treatments. The l-NAME challenge had a longer lasting effect on PAP than did the AG challenge. When both endothelial NOS and iNOS are inhibited by l-NAME, the ensuing reduction of NO synthesis leads to pulmonary arterial vasoconstriction (Martinez-Lemus et al., 1999, 2003; Wideman and Chapman, 2004). Wideman et al. (2005) reported that an l-NAME injection (40 mg) did not cause an increase in PAP within 5 min, but effectively amplified the pulmonary vasoconstriction elicited by a cellulose microparticle injection. The responsiveness of the birds to l-NAME in these experiments may be explained by the difference in dose (50 mg/kg of BW), the exposure to a cold temperature (which could have begun to create endothelial damage), and exposure to the previous challenges, reducing the availability of NO.
The MAP (Figure 2) showed the same tendencies as described for PAP. The first peak in MAP occurred within 30 s of the Epi challenge and returned to its basal measurement within 300 s after the Epi challenge. The MAP increased 60 s after the AG challenge and returned to its basal measurement within 120 s after the challenge in all groups. Sixty seconds after the l-NAME challenge, the MAP increased and returned to its basal measurement within 600 s in all groups. The MAP was not different among treatment groups at any sampling time. Heart rate was decreased in all groups after the Epi challenge and returned to its original measurements within 300 s in all groups (data not shown). Epinephrine triggers immediate increases in total peripheral resistance and pulmonary vascular resistance, which increase the MAP and PAP in spite of reductions in HR and cardiac output (Wideman, 1999). A decreased HR was also recorded after the AG and l-NAME challenges. Previous studies have shown similar observations for HR and MAP after an Epi or l-NAME challenge (Wideman, 1999; Wideman et al., 2005; Lorenzoni and Ruiz-Feria, 2006). In these experiments, neither MAP nor HR presented differences among treatments, and the time they took to return to basal measurements was similar among treatments, suggesting that the differences in PAP discussed above can be attributable to group differences in the pulmonary vascular tone.
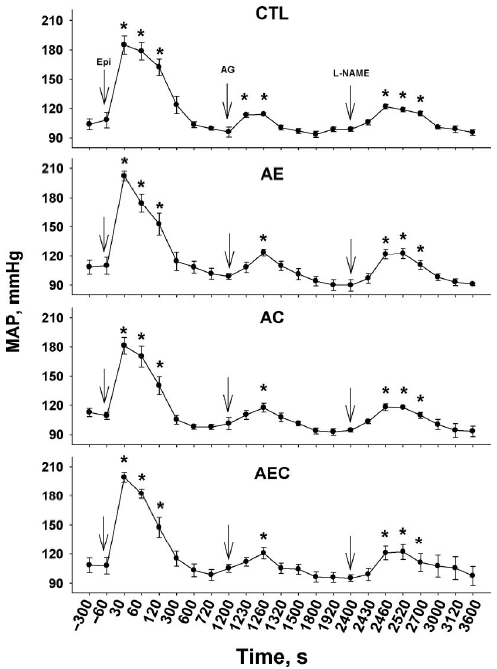
The in vivo half-life of NO is only a few seconds in dissolved biological fluids, where NO and reactive nitrogen species are rapidly converted into the stable oxidation products nitrite and nitrate (Dweik et al., 2001). Before the Epi challenge, we found that dietary supplementation of ARG in combination with antioxidant vitamins (AEC group) significantly increased the plasma concentrations of NO (nitrates and nitrites) in the blood, as compared with birds fed ARG and either one of the antioxidant vitamins (AE or AC groups) or a normal diet (CTL group; Table 1). In addition, before the Epi challenge, the NO concentrations between the AE and AC birds were not different, but were greater when compared with the NO concentrations of the CTL birds. These results strongly suggest that the better pulmonary vasodilatory capacity shown by the AEC birds after the Epi challenge (Figure 1) could be attributed to a greater availability of NO. Furthermore, birds showing intermediate cardiopulmonary performance after the Epi challenge (AE and AC birds) also had intermediate concentrations of NO metabolites in the blood, supporting the idea that VE and VC have complementary effects, protecting and enhancing the availability of NO, probably through reductions in oxidative stress. In addition to their antioxidant effects, VC and VE have been shown to improve the synthesis of endothelial- derived NO through the regeneration of tetrahydrobiopterin (an essential cofactor for NO synthesis) and the inhibition of protein kinase C activity, respectively (Carr et al., 2000). As expected, the concentration of plasma NO metabolites was reduced after the l-NAME challenge; however, even after the l-NAME challenge, birds in the AEC group had greater concentrations of NO than birds in the other treatments (experiments 1 and 2, and pooled data), further suggesting the beneficial effects of the concurrent supplementation of ARG, VE, and VC on NO availability.
At 3 wk of age (5 d after cold exposure began), the hematocrit value was greater in the CTL group than in the AEC group, but at wk 4, there were no differences among groups (Figure 3). However, at wk 5 and 6, hematocrit values were lowest (P < 0.05) in the AEC birds, and greatest in the CTL group. Hematocrit values for the AE and AC groups were similar, but lower when compared with the CTL birds, and greater when compared with the AEC birds (Figure 3). These hematocrit values were greater than those previously reported by Maxwell et al. (1992), but were similar to the ones we reported previously (Lorenzoni and Ruiz- Feria, 2006). A greater hematocrit value is associated with sustained hypoxia (Yersin et al., 1992) and has been shown to be correlated with ascites susceptibility (Wideman et al., 1998). The hematocrit values in the present experiments matched the results of cardiopulmonary performance and plasma NO concentrations, further supporting the role of ARG and antioxidants on the gas exchange rate and overall cardiopulmonary function in the AEC birds, followed by birds fed ARG and either VE or VC. The mortality attributed to ascites was numerically greater in the CTL group (9/48), followed by the AC group (8/48), the AE group (6/48), and the AEC group (4/48). The BW and RV:TV ratio were not different among treatments (data not shown). The results of the present experiments also indicated that the increase in H+ ions when arginine HCl was supplemented at amounts of 1 per cent (wt/wt) did not have any detrimental effect on the bird, and could be handled easily by the mechanisms of the bird to control the acid-base balance.
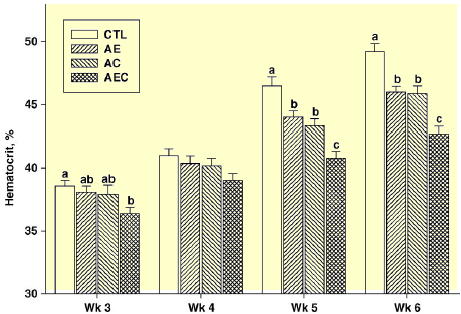
In summary, the concurrent supplementation of ARG, VE, and VC significantly improved the pulmonary vasodilation of broiler chickens grown under cold environmental conditions after an acute Epi challenge; the improved pulmonary vasodilation was associated with increased plasma NO concentrations and lower hematocrit concentrations. Thus, ARG, VE, and VC may have complementary effects on cardiopulmonary performance by increasing NO bioavailability through a greater substrate for NOS, and presumably by reducing the losses of NO associated with oxidative stress and reducing endothelial damage by NO-derived radicals. Further research is warranted to elucidate these mechanisms.
References
Baurhoo, B., L. Phillip, and C. A. Ruiz-Feria. 2007. Effects of purified lignin and mannan oligosaccharides on intestinal integrity and microbial populations in the ceca and litter of broiler chickens. Poult. Sci. 86:1070–1078.
Beckman, J. S., T. W. Beckman, J. Chen, R. Doan, P. A. Marshal, and B. A. Freeman. 1990. Apparent hydroxyl radical production by peroxynitrite: Implication for endothelial injury from nitric oxide and superoxide. Proc. Natl. Acad. Sci. USA 87:1620–1624.
Bottje, W., B. Enkvetchakul, and R. Moore. 1995. Effect of α-tocopherol on antioxidants, lipid peroxidation, and the incidence of pulmonary hypertension syndrome (ascites) in broilers. Poult. Sci. 74:1356–1369.
Bottje, W. G., G. F. Erf, T. K. Bersi, S. Wang, D. Barnes, and K. W. Beers. 1997. Effect of dietary dl-α-tocopherol on tissue α-and gamma tocopherol and pulmonary hypertension syndrome (Ascites) in broilers. Poult. Sci. 76:1506–1512.
Bottje, W. G., and R. F. Wideman. 1995. Potential role of free radicals in the pathogenesis of pulmonary hypertension syndrome. Poult. Avian Biol. Rev. 6:221–231.
Bowen, O. T., G. F. Erf, N. B. Anthony, and R. F. Wideman. 2006. Pulmonary hypertension triggered by lipopolysaccharide in ascites- susceptible and -resistant broilers is not amplified by aminoguanidine, a specific inhibitor of inducible nitric oxide synthase. Poult. Sci. 85:528–536.
Burton, R. R., E. L. Besch, and A. H. Smith. 1968. Effect of chronic hypoxia on the pulmonary arterial blood pressure of the chicken. Am. J. Physiol. 214:1438–1442.
Buys, N., C. W. Scheele, C. Kwakernaak, J. D. Van Der Klis, and E. Decuypere. 1999. Performance and physiological variables in broiler chicken lines differing in susceptibility to the ascites syndrome: 1. Changes in blood gases as a function of ambient temperature. Br. Poult. Sci. 40:135–139.
Carr, A. C., B. Z. Zhu, and B. Frei. 2000. Potential antiatherogenic mechanisms of ascorbate (vitamin C) and α-tocopherol (vitamin E). Circ. Res. 87:349–354.
Cueva, S., H. Sillau, A. Valenzuela, and H. Ploog. 1974. High altitude induced pulmonary hypertension and right heart failure in broiler chicken. Res. Vet. Sci. 16:370–374.
Dietert, R. R., and R. E. Austic. 1994. Environment–immune interactions. Poult. Sci. 73:1062–1076.
Dudgeon, S., D. P. Benson, A. MacKenzie, K. Paisley-Zyskiewicz, and W. Martin. 1998. Recovery by ascorbate of impaired nitric oxide-dependent relaxation resulting from oxidant stress in rat aorta. Br. J. Pharmacol. 125:782–786.
Dweik, R. A., S. A. Comhair, B. Gaston, F. B. Thunnissen, C. Farver, and M. J. Thomassen, M Kavuru, J., H. M. Hammel, Abu- Soud, and S. C. Erzurum. 2001. Nitric oxide chemical events in the human airway during the immediate and late antigen induced asthmatic response. Proc. Natl. Acad. Sci. USA 98:2622–2627.
Enkvetchakul, B., W. Bottje, N. Anthony, R. Moore, and W. Huff. 1993. Compromised antioxidant status associated with ascites in broilers. Poult. Sci. 72:2272–2280.
Fresquet, F., F. Pouraqeaud, V. Leblais, R. P. Brandes, J. P. Savineau, R. Marthan, and B. Muller. 2006. Role of reactive oxygen species and gp91phox in endothelial dysfunction of pulmonary arteries induced by chronic hypoxia. Br. J. Pharmacol. 148:714–723.
Grobe, A. C., S. M. Wells, E. Benavidez, P. Oishi, A. Azakie, J. R. Fineman, and S. M. Black. 2006. Increased oxidative stress in lambs with increased pulmonary blood flow and pulmonary hypertension: Role of NADPH oxidase and endothelial NO synthase. Am. J. Physiol. 290:L1069–L1077.
Hishikawa, K., and T. F. Lüscher. 1997. Pulsatile stretch stimulates superoxide production in human aortic endothelial cells. Circulation 96:3610–3616.
Jandel Scientific. 1994. SigmaStat Statistical Software User’s Manual. Jandel Scientific Software, San Rafael, CA.
Jung, O., S. L. Marklund, H. Geiger, T. Pedrazzini, R. Busse, and R. P. Brandes. 2003. Extracellular superoxide dismutase is a major determinant of nitric oxide bioavailability: In vivo and ex vivo evidence from ecSOD-deficient mice. Circ. Res. 93:622–629.
Keller, U., C. Brandsch, and K. Eder. 2004. Supplementation of vitamins C and E increases the vitamin E status but does not prevent the formation of oxysterols in the liver of guinea pigs fed an oxidised fat. Eur. J. Nutr. 43:353–359.
Ladmakhi, M. H., N. Buys, E. Dewil, G. Rahimi, and E. Decuypere. 1997. The prophylactic effect of vitamin C supplementation on broiler ascites incidence and plasma thyroid hormone concentration. Avian Pathol. 26:33–44.
Liu, J. F., and Y. W. Lee. 1998. Vitamin C supplementation restores the impaired vitamin E status of guinea pigs fed oxidized frying oil. J. Nutr. 128:116–122.
Lopez-Lopez, J. G., F. Perez-Vizcaino, A. L. Cogolludo, M. Ibarra, F. Zaragoza-Arnaez, and J. Tamargo. 2001. Nitric oxide and nitric oxide donors-induced relaxation and its modulation by oxidative stress in piglet pulmonary arteries. Br. J. Pharmacol. 133:615–624.
Lorenzoni, A. G., and C. A. Ruiz-Feria. 2006. Effects of vitamin E and l-arginine on Cardiopulmonary function and ascites parameters in broilers chickens reared under sub-normal temperatures. Poult. Sci. 85:2241–2250.
Martinez-Lemus, L. A., R. K. Hester, E. J. Becker, J. S. Jeffrey, and T. W. Odom. 1999. Pulmonary artery endothelium-dependent vasodilation is impaired in a chicken model of pulmonary hypertension. Am. J. Physiol. 277:R190–R197.
Martinez-Lemus, L. A., R. K. Hester, E. J. Becker, G. A. Ramirez, and T. W. Odom. 2003. Pulmonary artery vasoactivity in broiler and Leghorn chickens: An age profile. Poult. Sci. 82:1957– 1964.
Maxwell, M. H., and G. W. Robertson. 1998. UK survey of broiler ascites and sudden death syndromes in 1993. Br. Poult. Sci. 39:203–215.
Maxwell, M. H., G. W. Robertson, and C. C. McCorquodale. 1992. Whole blood and plasma viscosity values in normal and ascitic broiler chickens. Br. Poult. Sci. 33:871–877.
McQueston, J. A., D. N. Cornfield, I. F. McMurtry, and S. H. Abman. 1993. Effects of oxygen and exogenous l-arginine on EDRF activity in fetal pulmonary circulation. Am. J. Physiol. 264:H865–H871.
Nespereira, B., M. Pérez-Ilzarbe, P. Fernández, A. M. Fuentes, J. A. Páramo, and J. A. Rodríguez. 2003. Vitamins C and E downregulate vascular VEFG and VEGFR-2 expression in apolipoprotein- E-deficient mice. Atherosclerosis 171:67–73.
NRC. 1994. Nutrient Requirements of Poultry. 9th rev. ed. Natl. Acad. Press, Washington, DC.
Palmer, L. A., G. L. Semenza, M. H. Stoler, and R. A. Johns. 1998. Hypoxia induces type II NOS gene expression in pulmonary artery endothelial cells via HIF-1. Am. J. Physiol. 274:L212– L219.
Pardue, S. L., and J. P. Thaxton. 1986. Ascorbic acid in poultry: A review. World’s Poult. Sci. J. 42:107–123.
Peacock, A. J., C. Pickett, K. Morris, and J. T. Reeves. 1990. Spontaneous hypoxemia and right ventricular hypertrophy in fast growing broiler chickens reared at sea level. Comp. Biochem. Physiol. A 97:537–541.
Rodriguez-Gomez, I., R. Wangensteen, J. M. Moreno, V. Chamorro, A. Osuna, and F. Vargas. 2005. Effects of chronic inhibition of inducible nitric oxide synthase in hyperthyroid rats. Am. J. Physiol. Endocrinol. Metab. 288:E1252–E1257.
Ruiz-Feria, C. A., M. T. Kidd, and R. F. Wideman. 2001. Plasma levels of arginine, ornithine, and growth performance of broilers fed supplemented l-arginine during cool temperature exposure. Poult. Sci. 80:358–369.
Ruiz-Feria, C. A., Y. Yang, and H. Nishimura. 2004. Do incremental increases in blood pressure elicit neointimal plaques through endothelial injury? Am. J. Physiol. 287:R1486–R1493.
Sahin, K., N. Sahin, and S. Yaralioglu. 2002. Effects of vitamin C and vitamin E on lipid peroxidation, blood serum metabolites and mineral concentrations of laying hens reared at high ambient temperature. Biol. Trace Elem. Res. 85:35–45.
Scheele, C. W., W. De Wit, M. T. Frankenhuis, and P. F. Vereijken. 1991. Ascites in broilers. 1. Experimental factors evoking symptoms related to ascites. Poult. Sci. 70:1069–1083.
Schneider, C. 2005. Chemistry and biology of vitamin E. Mol. Nutr. Food Res. 49:7–30.
Schroeder, R. A., A. Ewing, J. V. Sitzmann, and P. C. Kuo. 2000. Pulmonary expression of iNOS and HO-1. protein is upregulated in a rat model of prehepatic portal hypertension. Dig. Dis. Sci. 45:2405–2410.
Smith, F. M., N. H. West, and D. R. Jones. 2000. The cardiovascular system. Pages 141–231 in Sturkie’s Avian Physiology. 5th ed. G. C. Whittow, ed. Academic Press, San Diego, CA.
Szabo, C. 1996. The pathophysiological role of peroxynitrite in shock, inflammation and ischemia-reperfusion injury. Shock 6:79–88.
Tamir, H., and S. Ratner. 1963. A study of ornithine, citrulline and arginine synthesis in growing chicks. Arch. Biochem. Biophys. 102:259–269.
Tan, X., W. D. Sun, J. C. Li, J. Q. Pan, Y. J. Liu, J. Y. Wang, and X. L. Wang. 2005. l-Arginine prevents reduced expression of endothelial nitric oxide synthase (NOS) in pulmonary arterioles of broilers exposed to cool temperatures. Vet. J. 173:151–157.
Taylor, R. L., R. E. Austic, and R. Dietert. 1992. Dietary arginine influences Rous sarcoma growth in a major histocompatibility B complex progressor genotype. Proc. Soc. Exp. Biol. Med. 199:38–41.
Teshfam, M., G. H. Nikbakht, N. Brujeni, and H. Hassanpour. 2006. Evaluation of endothelial and inducible nitric oxide synthase mRNA expression in the lung of broiler chickens with developmental pulmonary hypertension due to cold stress. Br. Poult. Sci. 47:223–229.
Walton, J. P., R. J. Julian, and E. J. Squires. 2001. The effects of dietary flax oil and antioxidants on ascites and pulmonary hypertension in broilers using a low temperature model. Br. Poult. Sci. 42:123–129.
Wideman, R. F. 1988. Ascites in Poultry. Monsanto Nutr. Update 6:1–7.
Wideman, R. F. Jr. 1999. Cardiac output in four-, five- and sixweek- old broilers, and hemodynamic responses to intravenous injections of epinephrine. Poult. Sci. 78:392–403.
Wideman, R. F., O. T. Bowen, G. F. Erf, and M. E. Chapman. 2006. Influence of aminoguanidine, an inhibitor of inducible nitric oxide synthase, on the pulmonary hypertensive response to microparticle injections in broilers. Poult. Sci. 85:511–527.
Wideman, R. F., and M. E. Chapman. 2004. N-Nitro-l-arginine methyl ester (l-NAME) amplifies the pulmonary hypertensive response to endotoxin in broilers. Poult. Sci. 83:485–494.
Wideman, R. F., G. F. Erf, and M. E. Chapman. 2005. N-Nitro-larginine methyl ester (l-NAME) amplifies the pulmonary hypertensive response to microparticle injections in broilers. Poult. Sci. 84:1077–1091.
Wideman, R. F., Y. K. Kirby, M. Ismalil, W. Bottje, R. Moore, and R. Vardeman. 1995. Supplemental l-arginine attenuates pulmonary hypertension syndrome (ascites) in broilers. Poult. Sci. 74:323–330.
Wideman, R. F., Y. K. Kirby, C. D. Tackett, N. E. Marson, and R. W. Mcnew. 1996. Cardio-pulmonary function during acute unilateral occlusion of the pulmonary artery in broilers fed diets containing normal or high levels of arginine-HCL. Poult. Sci. 75:1587–1602.
Wideman, R. F., and C. D. Tackett. 2000. Cardio-pulmonary function in broilers reared at warm or cool temperatures: Effect of acute inhalation of 100% oxygen. Poult. Sci. 79:257–264.
Wideman, R. F. Jr., T. Wing, Y. K. Kirby, M. F. Forman, N. Marson, C. D. Tackett, and C. A. Ruiz-Feria. 1998. Evaluation of minimally invasive indices for predicting ascites susceptibility in three successive hatches of broilers exposed to cool temperatures. Poult. Sci. 77:1565–1573.
Xiang, R. P., W. D. Sun, J. Y. Wang, and X. L. Wang. 2002. Effect of vitamin C on pulmonary hypertension and muscularisation of pulmonary arterioles in broilers. Br. Poult. Sci. 43:705–712.
Yersin, A. G., W. E. Huff, L. F. Kubena, M. H. Elissalde, R. B. Harvey, D. A. Witzel, and L. E. Giroir. 1992. Changes in hematological, blood gas, and serum biochemical variables in broilers during exposure to simulated high altitude. Avian Dis. 36:189–196.