Effects of conventional and novel processing on the feed value of canola meal for poultry
By H L Classen, R W Newkirk and D D Maenz, University of Saskatchewan - The desolventisation/toasting stage of pre-press solvent extraction was found to reduce the digestible amino acid content of canola meal in broilers. In addition, inconsistency in this stage of processing was also shown to be responsible for variability in this trait.Summary
Although toasting reduces the levels of toxic glucosinolates, broilers fed toasted meal had reduced performance in comparison to birds fed non-toasted meal. Neutral detergent insoluble nitrogen in canola meal samples proved to give a reasonable estimate of meal quality. In vitro experiments demonstrated that elevated temperature and meal moisture during desolventisation/toasting were responsible for protein damage. In conclusion, toasting that occurs during conventional pre-press solvent extraction can cause detrimental effects on canola meal quality and may not be necessary for the production of meal for poultry.
I. INTRODUCTION
The objectives of this paper are to discuss various aspects of processing that can influence the nutritional value of canola meal and to describe an alternate method of processing that has potential to increase the utilisation of canola nutrients. To set the stage for this discussion, it is appropriate to briefly describe the Canadian canola industry and the factors that led to increased research in the area of canola processing.
Rapeseed was introduced into Canada as a crop in the 1940's as a method of diversifying crop production, particularly in the prairie provinces. Production was initially designed to provide oil for industrial rather than food purposes and the seed was characterised by high levels of erucic acid (23-45% of oil) and glucosinolates (120 to 150 µmol/g total aliphatic glucosinolates on an oil free, dry basis). Post World War II, the demand for rapeseed declined dramatically and alternate uses were pursued. One of the major success stories of Canadian agricultural research was the reduction of erucic acid and aliphatic glucosinolates to less than 2% and 30 µmol/g of oil free meal, respectively. To identify this improved version of rapeseed, the term canola was introduced. This has subsequently been internationally recognised as a name for "double zero" cultivars of rapeseed. Canola is by far the most important oilseed grown in Canada and production has ranged from 5 to 9 million tonnes in recent years.
Approximately 50% of seed is processed in Canada with the remainder exported. The products of Canadian processing are used within country or exported, with the majority of exports being to the United States.
The meal derived from canola seed is marketed as a feed ingredient to a wide range of animal species including chickens, turkeys, pigs and cattle. For poultry, canola meal is considered to have moderate to high protein level (34-39%), good amino acid balance, a relatively low apparent metabolisable energy (AME) and high levels of phytate (3%).
Low and variable amino acid digestibility (NRC, 1994) are also characteristic of canola meal and may be responsible for its relatively lower than expected value (55-65%) in relationship to soybean, despite having approximately 75% of the protein content. The low price of canola meal in some markets, and poor and variable amino acid digestibility in non-ruminant species led to research on the effect of processing on the nutritional value of canola meal for broiler chickens. An alternate method of processing was also investigated that results in more effective utilisation of canola nutrients by a wider range of species.
II. CANOLA PROCESSING
Canola seed in Canada is virtually all processed using pre-press solvent extraction. This procedure uses screw presses to extract a portion of the oil and then removes the remainder of the oil by solvent extraction. An example of pre-press solvent processing of canola seed is shown in Figure 1. The initial step in processing is the removal of major non-canola seed materials (screenings, often added back to meal after processing). The seed is then pre-conditioned by drying (to 6-7% moisture) and heated to 75-78°C to prevent seed shattering and improve processing. Flaking then ruptures the seed coat and some oil cells prior to cooking (75-85°C for 20 to 60 min).
The latter step denatures hydrolytic enzymes such as myrosinase and further ruptures oil cells. Destruction of myrosinase is essential to prevent hydrolysis of glucosinolates to more toxic and undesirable sulphur compounds. Pressure expelling then removes from 60 to 70% of the oil prior to solvent extraction with hexane. Meal exiting hexane extraction has low levels of oil and is laden with hexane (35%). The meal then enters the desolventisation/toasting (DT) stage of processing which uses a vertical column with multiple trays to heat the meal. Hexane is evaporated from the meal as a result of the indirect heat of the heated trays as well as by direct heat from the injection of steam (sparge steam) into the meal in the final lower trays. The temperature increases as meal proceeds from tray to tray, being relatively low at higher trays because of hexane evaporation but reaching temperatures of 100 to 110°C in the final trays due to steam injection.
Condensation of steam increases the meal moisture content to 16-18%. Moisture can also enter the DT stage via water sprayed on the upper tray to control dust and water found in gums that may be returned to the meal at this stage. This stage also "toasts" the meal to reduce the level of anti-nutritional glucosinolates and possibly other undefined factors. The meal is then dried and cooled, and possibly ground and pelleted.
- Effect of processing stage on nutritional value of meal
In order to study the impact of various stages of processing on meal nutritional quality, samples were collected on three separate occasions from a single commercial processing plant. On each collection, samples were collected after six stages as outlined in Figure 1 and then used to study meal nutrient content as well as ileal apparent amino acid digestibility and fecal AME using broiler chicks (Newkirk et al., 2003a). With the exception of AME changes associated with oil extraction, only minor effects on nutritional value were seen up to and including solvent extraction. However, DT reduced the lysine (LYS) content of the meal (6.03 to 5.50% of crude protein) and the digestibility of all amino acids except for alanine (ALA), glycine (GLN), serine (SER) and tyrosine (TYR). Of particular interest was the digestibility of LYS which decreased from 87 to 79%. In addition the coefficient of variability for LYS digestibility increased from 1.4% before to 5.6% after DT, supporting the concept that this stage of processing is not only responsible for decreasing the digestible amino acid content but also increasing the variability. Meal AME decreased as oil was removed during the expelling and solvent extraction phases and there was a non-significant reduction after the DT stage. Canola meal coloration also was affected by stage of processing with meal being yellow with black hull flecks and dark brown prior to and after DT, respectively. Maillard and protein-polyphenolic reactions are likely responsible for meal darkening.
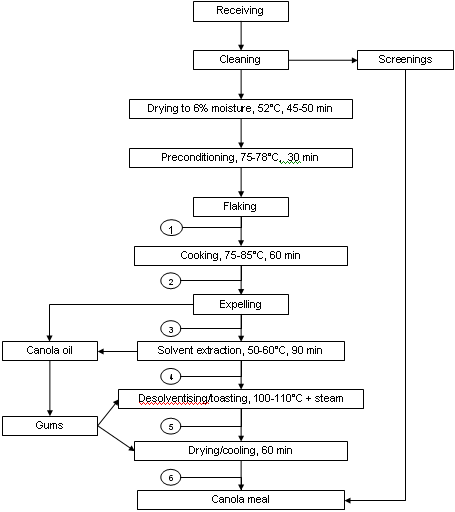
Figure 1. Example of pre-press solvent extraction of canola seed.
Circled numbers represent points of sample collection for evaluation
of amino acid availability (Newkirk et al., 2003a)
A second experiment was completed to verify the impact of DT on meal quality in seven western Canadian processing plants and also study the effect of this process on glucosinolate content (Newkirk et al., 2003b). Samples were collected before (non-toasted canola meal - NTCM) and after DT (toasted canola meal - TCM) at the same time to reduce the potential for variability due to seed source. Samples were collected on five separate occasions from each of five plants while only a limited number of samples were available from the remaining two processors. NTCM samples were air desolventised prior to testing. Prior to DT, samples were relatively uniform in amino acid content and digestibility but as had been shown in the previous study, DT reduced the content and/or digestibility of amino acids in the meal to varying degrees.
As a consequence, DT reduced the digestible content of all amino acids measured (not including TYR and TRP) with the exception of GLU. The total digestible amino acid content of the TCM samples (69.6g/16 g N; range 56.6-75.6) was on average 12% lower than for the NTCM samples (77.6 g/16g N; range 73.1-82.0). As expected, LYS was affected more than other amino acids. The average LYS content and apparent digestibility of NTCM samples was 6.0 g/16g N (range 5.7-6.3) and 89.75 (range 87-92%), respectively. After DT, LYS content and digestibility values were 5.6 g/16 g N (range 5.3-5.9) and 78.6% (range 65.5-85.7%), respectively. Significant effects of processing plant, and interactions between processing plant and DT demonstrated that considerable variability occurs both between and within plants. This is demonstrated by LYS digestibility values for all collected samples (26 non-toasted and 31 toasted) which are shown in Figure 2. DT reduced the levels of aliphatic glucosinolates from 10.5 (range 7.8-15.1) to 6.16 (range 1.2-12.5) mol/g meal. These are well below the maximum standard set for canola meal and the nutritional significance of these levels remains to be determined but is likely low.
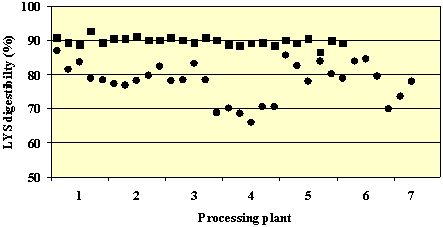
Figure 2. Apparent LYS digestibility (%) of non-toasted (¦ NTCM, n=26) and toasted (? NTCM, n=31) canola meal samples
In conclusion, the data from these experiments demonstrate the importance of DT in determining the nutritional value of canola meal, both in terms of level and variability in digestible amino acid content. The negative effects of this stage of canola processing are likely related to the temperature and moisture content of the meal during this stage and the length of time to which the meal is exposed to these conditions. The loss of content and digestibility can be affected by a number of reactions including various stages of Maillard reactions, protein/polyphenolic reactions and protein/protein reactions. Maillard reactions are likely predominant and have been broken down into early, advanced and final reactions (Mauron, 1981). Final reactions result in decomposition of involved amino acids and as such would not be detected by amino acid analysis. Products of early and advanced reactions would be measured but would be unavailable for digestion. This corresponds with our results in terms of reduced amino acid content and reduced apparent digestibility, and suggests that various stages of Maillard reactions are occurring during DT.
III. NON-TOASTED AND TOASTED CANOLA MEAL IN BROILER DIETS
Despite the apparent nutritional advantage of non-toasted vs. toasted meal in terms of digestible amino acid content, there may be other advantages of DT that would become evident in an animal feeding trial. To provide samples to allow this comparison, NTCM and TCM samples were obtained from a commercial processor on the same day to minimise differences in originating seed (Newkirk and Classen, 2002). The NTCM sample was desolventised using a pilot scale desolventiser/toaster equipped with two steam heated trays but without the addition of steam directly into the meal. The rate of flow through the desolventiser/toaster was adjusted so that the exit temperature was approximately 100°C. It was hypothesised that the use of low heat application and no moisture being added in the form of sparge steam would result in meal that was not toasted or toasted only to a limited extent. The final meals were compared on the basis of colour (NTCM - yellow, TCM - light brown), and neutral detergent insoluble nitrogen (% of N; NTCM - 11.31, TCM - 19.73) and aliphatic glucosinolate content ( mol/g; NTCM - 11.52, TCM - 7.84). The results confirm that the NTCM was not toasted and suggest that the TCM was relatively mildly toasted.
Commercial broilers (total of 3240) were used to compare the feeding value of the NTCM and TCM meals. Meal samples replaced 0, 20, 40, 60, 80 and 100% of the soybean meal in a wheat-soybean meal based diet with diets formulated to be iso-energetic and contain the same levels of digestible LYS, methionine (MET) and cystine (CYS), and arginine (ARG). Overall, growth rate was superior for birds fed the NTCM (2.181 vs 2.148 kg at 39 days of age) as was gain to feed ratio from 0 to 19 days of age (0.642 vs 0.637). For both meal types growth rate responded in a quadratic fashion to the level of addition. Up to at least 60% soybean meal replacement produced performance equal to the control diet while higher levels of inclusion resulted in decreased growth. Feeding TCM increased proportional liver weight in comparison to NTCM.
A significant interaction between meal type and level of inclusion showed that as diet level of TCM increased, proportional heart weight also increased. Similarly, serum T3 levels increased in a linear fashion as levels of TCM increased in the diet but were unaffected by level of NTCM. Overall, NTCM was marginally superior to a mildly toasted canola meal as a feed ingredient for broiler chickens. This suggests that non-toasted canola meal with higher levels of digestible amino acids could be fed to poultry with no detrimental effect. Improved performance in chickens fed NTCM may be due to its enhanced digestible nutrient content, but the effects of TCM on liver and heart weight, and serum T3 indicate that toasting may produce an undefined toxic factor(s). Before elimination of toasting can be recommended, its effects on feeding value must be studied in other species that may have different sensitivities to palatability and other factors in the meal.
IV. ASSESSING CANOLA MEAL QUALITY
Soybean meal processing is required to destroy anti-nutritional factors and the optimum conditions are well defined. Relatively simple assays have also been developed to assist processors and feed manufacturers in assessing both under (urease activity) and over processing (solubility in 0.2% potassium hydroxide) of the meal. The finding that canola meal processing causes variable and low digestible amino acid levels demonstrates a similar need to monitor meal quality from both a processor and meal user standpoint.
To date protein solubility has received most attention and solubility does decrease in response to heat application (Anderson-Hafermann et al., 1993; Pastuszewska et al., 1998). However, there is little information on how it is related to amino acid content and digestibility. Regression analysis from a limited data set from our laboratory has indicated that neutral detergent insoluble nitrogen (NDIN) may also be able to predict LYS digestibility. NDIN is commonly used to assess feed quality for ruminant species and it appears that protein that remains insoluble during neutral detergent extraction is not or poorly digestible by chickens. Near infrared reflectance spectroscopy (NIRS) is able to estimate the moisture, protein and amino acid content of feed ingredients (Williams et al., 1983; Fontaine et al., 2001), and appears also have potential in assessing nutrient digestibility (van Kempen, 1998). Therefore, NIRS may also have merit in assessing the nutritional value of canola meal.
Research was conducted to assess the relationship of in vitro analyses and amino acid digestibility (Newkirk and Classen, 2001). Protein solubility in 0.5% KOH, NDIN and NIRS were used to establish relationships using the samples collected from the western Canadian processing plants discussed above. With the exception of NIRS, regressions analyses were completed separately for all samples (NTCM and TCM) and for those which were conventionally processed (TCM). Using all samples, a linear relationship was found between LYS digestibility (R2=0.65) and digestible LYS content (R2=0.74), and protein solubility in 0.5% KOH. However, if only TCM samples were included in the analyses, the relationship was weak (R2=0.17). Therefore protein solubility in KOH is not sufficiently accurate to predict the nutritional value of canola meal. NDIN was negatively associated with the digestibility and digestible content of LYS (R2=0.78) and other essential amino acids when all samples were included in the analysis. Even with only TCM samples, the relationships for LYS digestibility (R2=0.54) and digestible LYS content (R2=0.58) were sufficiently strong to have value in quality assessment of canola meal. Based on this study, it would appear that meals with less than 12% of the protein as NDIN are of very good nutritional value.
NIRS accurately predicted both LYS digestibility (R2=0.92) and digestible content of LYS (R2=0.84) when both NTCM and TCM samples were used. Unfortunately there was no opportunity to evaluate only TCM samples to determine the predictive value of NIRS using conventionally processed canola meal. Additional research is required to establish if it has value in assessing canola meal quality. Meal colour has also been mentioned a number of times in this paper as a character that distinguishes NTCM and TCM samples. However, it has not been possible to use colour as an accurate predictor, possibly because early Maillard reactions reduce amino acid digestibility without causing colour reactions. In conclusion, NDIN is the best predictor of canola meal quality at the present time but NIRS has potential to be used in the future. Because of the expense of biological testing required for calibration and validation of NIRS, it may be useful to use NIRS to indirectly predict quality by estimating NDIN content.
V. PROCESSING FACTORS AFFECTING MEAL QUALITY
It was of interest to study the conditions that are associated with reduced content and availability of amino acids as a result of DT. Based on the knowledge of Maillard reactions in other processed material, time of exposure to various temperature and moisture conditions was considered to be the most relevant for study. Therefore, air desolventised post-hexane extraction meal was exposed to various temperature and moisture conditions in a laboratory setting.
In the first experiment, meal was exposed (0-60 min) to temperatures ranging from 85 to 120 C using an isothermal autoclave. It was not possible to regulate moisture content in this experiment or accurately assess the moisture level after removal from the autoclave. At temperatures less than 100 C there was no change in NDIN regardless of time of exposure.
Exposure to higher temperatures resulted in a quadratic increase in NDIN with the degree of response temperature dependent. It was concluded that temperatures less than 100 C during desolventisation were desirable to prevent protein damage.
The effect of temperature and moisture were examined in a second experiment using NDIN, amino acid content and colour as response criteria. Temperature and moisture content ranged from 100 to 115 C and 7 to 18%, respectively, and meal was treated for 10 min. Moisture affected NDIN, amino acid content and colour of meal in a temperature related manner. At 7% moisture no effects were noted regardless of temperature, while with increasing moisture content the levels of NDIN increased, amino acid levels decreased (particularly LYS) and the meal became darker with increased temperature. The effects of moisture and temperature on NDIN are demonstrated in Figure 3. The results indicate that moisture is an important factor determining protein damage during canola meal desolventisation. The moisture level of meal exiting solvent extraction is approximately 7%.
In conventional processing, moisture is added via direct injection of steam, water contained in gums that could enter at this stage, and water used to control dust in the upper tray of the desolventiser/toaster. Although it would be desirable to minimise moisture addition, other factors may delay conversion of processing plants. In the processing plants currently in use in Canada, the steam added in the final plates of the desolventiser/toaster assist in driving residual hexane though upper trays and meal prior to exiting the top of the unit. Alternate methods of removing hexane at this stage are required before sparge steam can be eliminated. Large capital investments in existing equipment will also slow adoption but alternative methods of desolventisation should be developed for the next generation of processing equipment.
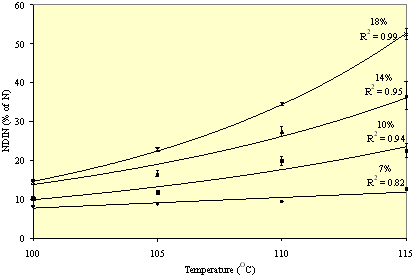
Figure 3. The effect of moisture and temperature on the neutral detergent insoluble nitrogen content (NDIN, as a proportion of N) of canola meal (± standard error) after 10 minutes of heating
VI. NOVEL PROCESSING OF CANOLA MEAL
Canola meal is a composite of nutrients/materials that make it less than ideal for the animals to which it is fed. Canola meal has an excellent amino acid profile and its protein has low antigenicity, but high levels of fibre, phytate and possibly other anti-nutritional factors restrict it use in poultry and swine, and eliminates its potential in aquaculture. In contrast, ruminants can utilise more of the fibre and phytate phosphorus, but the high quality amino acids are an expensive source of nitrogen for rumen bacteria. As such, canola meal is non-optimised for use as a feed ingredient. This knowledge has led to research investigating the economic fractionation of canola meal into value added products that more closely match the requirements of target species. In turn, a technology company was developed that is currently entering the commercialisation phase (http://www.mcnbioproducts.com/).
The fractionation procedures are proprietary, but in general the process involves liquid fractionation. Although it is possible to obtain a number of different product lines, the
three of most interest are insoluble, soluble and fibre protein fractions. The insoluble and soluble products maintain the excellent amino acid balance and low antigenicity characteristics of canola meal but concentrate the protein (650 to 800 g/kg and eliminate or reduce the levels of phytate and other anti-nutritional factors. These products are designed to compete with fish meal and high protein plant concentrates in non-ruminant and aquaculture diets. The fibre protein product retains high quality insoluble protein (34%) and is primarily designed for ruminant markets. A fourth stream from this processing system is sugar rich and contains high levels of available minerals. It is envisaged that this product may be combined with the fibre protein as a ruminant feedstuff.
The direction taken for this type of technology represents an opportunity for other feed ingredients that are undervalued and non-optimised for animal feeding. By following similar processing methods, the resulting products have the potential to generate value in excess of that derived from the original commodity. Methods of adding value to feed ingredients using new process technology are undoubtedly going to see increased prominence with more pressure for both economic and environmentally sustainable animal production.
REFERENCES
Anderson-Hafermann, J. C., Zhang, Y. and Parsons, C. M. (1993). Poultry Science, 72:326-333.
Fontaine, J., Hoerr, J. and Schirmer, B. (2001). Journal of Agricultural and Food Chemistry, 49:57-66.
Mauron, J. (1981). Progress in Food and Nutrition Science, 5:5-35.
Newkirk, R.W. and Classen, H.L. (2001). Proceedings of the 22nd Western Nutrition Conference - Pre-Conference Symposium pp 25-35, September 25-27, Saskatoon, SK.
Newkirk, R.W. and Classen, H.L. (2002). Poultry Science, 81:815-825.
Newkirk, R.W., Classen, H.L. and Edney, M.J. (2003a). Animal Feed Science and Technology, 104:111-119.
Newkirk, R.W., Classen, H.L., Scott, T.A. and Edney, M.J. (2003b). Canadian Journal of Animal Science, 83:131-139.
NRC (1994). Nutrient Requirements of Poultry. National Academy Press, Washington D.C.
Pastuszewska, B., Buraczewska, L., Ochtabinska, A. and Buraczewski, S. (1998). Journal of Animal Feed Science, 7:73-82.
van Kempen, T. C. B. J. (1998). Animal Feed Science and Technology, 76:139-147.
Williams, P.C., Norris, K.H., Gehrke, C.W. and Bernstein, K. (1983). Cereal Foods World, 28:149-152.
Source: University of Saskatchewan - Taken from website - March 2005