Estimation of Genetic Parameters for Fat Deposition and Carcass Traits in Broilers
By S. Zerehdaran and J. A. M. van Arendonk, Wageningen Institute of Animal Science; A. L. J. Vereijken, Nutreco Breeding Research Center and E. H. van der Waaij, University of Utrecht - Abdominal and subcutaneous fat are regarded as the main sources of waste in the slaughterhouse.
Abstract
Fat stored intramuscularly is regarded a favorite trait related to meat quality. The objective of current study was to estimate genetic parameters for fat deposition in the 3 different parts of body and their relationships with other carcass traits. Traits were recorded for 1,752 females and 1,526 males from a meat-type chicken line. Heritability estimates for abdominal fat percentage, skin percentage as a measure of subcutaneous fat, and intramuscular fat percentage were 0.71, 0.24, and 0.08, respectively. Heritabilities of the other carcass traits were moderate to high (0.28 to 0.73).
There was a high genetic correlation between abdominal fat weight and skin weight (0.54), whereas the genetic correlation between abdominal fat weight and intramuscular fat percentage was almost zero (0.02). The BW at 7 wk showed a positive genetic correlation with fat production traits, which were high for intramuscular fat percentage (0.87) and moderate for skin percentage (0.17) and abdominal fat percentage (0.13). Therefore carcass traits could be improved by selection for increased breast muscle and reduced abdominal fat without decreased intramuscular fat.
Introduction
The primary goal of broiler breeding is to improve profitability of broiler meat production. Until recently most birds were sold whole, but there has been a dramatic increase in the proportion of birds being grown for portioning and further processing (Ewart, 1993). Poultry production and processing technologies have become rapidly accessible and are being implemented on a worldwide basis, which will allow continued expansion and competitiveness in this meat sector (Aho, 2001). Therefore, the success of poultry meat production has been strongly related to improvements in growth and carcass yield, mainly by increasing breast proportion and reducing abdominal fat. Intensive selection of meat-type chickens for growth for more than 50 yr has increased growth rate but rapid growth has been accompanied by a number of negative consequences, including an increase in fat deposition (Griffin, 1996).
Abdominal and subcutaneous fat are being regarded as the main sources of waste in the slaughterhouse. Because abdominal fat is highly correlated (0.6 to 0.9) with total carcass lipids, it is used as the main criterion reflecting excessive fat deposition in broilers (Chambers, 1990). Havenstein et al. (2003) described that fat in broiler (at 43 d of age) accounts for as much as 10 to 15% of the total carcass weight. Therefore, there is substantial potential to improve feed efficiency and carcass quality by further reducing fatness. Besides abdominal and subcutaneous fat that are unfavorable traits, intramuscular fat could be a favorable trait. In beef cattle, intramuscular fat makes some contribution to sensory palatability.
Measures of sensory palatability incorporate attributes such as tenderness, juiciness, and flavor (Oddy et al., 2001). Nishimura et al. (1999) reported that intramuscular fat in longissimus muscle may physically alter connective tissue structure and thereby reduce toughness of the meat. Although intramuscular fat plays a major role in broiler meat quality (flavor and juiciness) (Chizzolini et al., 1999), very few estimates of its heritability and genetic correlation with other important traits have been published. The speed of feathering in birds can also influence carcass composition. Fotsa et al. (2001) mentioned, owing to the considerable power of thermal insulation of the plumage, this phenomenon may favor heat dissipation into the environment and thus has an influence on traits of economic importance (i.e., feed intake, growth rate, and fatness).
The possibility of genetically improving carcass quality by selection depends on the genetic variability of BW and body composition. Body composition can be significantly improved by selection, as shown by the level of breast muscle heritability ranging from 0.53 and 0.65 in the studies of Vereijken (1992), Le Bihan-Duval et al. (1998, 1999), and Rance et al. (2002). For abdominal fat, heritability ranges between 0.50 and 0.80 (Chambers, 1990; Griffin et al., 1991; Le Bihan-Duval et al., 1998; Rance et al., 2002). The objective of current study was to estimate heritabilities and genetic and phenotypic correlations for fat deposition in 3 different parts of the carcass with other carcass traits.
MATERIALS AND METHODS
Population Two genetically different outcross broiler dam lines originating from the White Plymouth Rock breed were chosen as the foundation of the experimental population. The maternal line had a rather high reproductive performance and was fast feathering, and the paternal line had a rather high growth performance and was slow feathering. After 9 generations of intercrossing (F9), carcass-related traits were recorded for 3,278 birds (i.e., 1,752 females and 1,526 males). These birds were produced by 31 sires and 57 dams. The total pedigree file consisted of 13,491 birds. The experimental birds were hatched during 13 different wk in 1999 and 2000. Birds were housed in a litter system for broilers until the age of 7 wk. The birds were in the same pen starting from d 0, where they received feed and water ad libitum. Animal density was around 20 birds/m2, and illumination was 23 h/d. A commercial broiler feed, consisting of crumbled concentrates and containing 2,980 kcal/kg and 21% protein, was used.
Traits
Body weights at 5 and 7 wk of age (BW5 and BW7) were measured on live birds; BW7 was measured after 4 h with no access to feed and prior to transporting the birds for processing. After slaughter at the same day of age, the weights of carcass (CW), abdominal fat (AFW), breast muscle (BMW), and skin (SW) were measured. The CW was measured on the chilled carcass after removal of feathers, head, lungs, liver, kidneys, gastrointestinal tract, and abdominal fat. The ratio of these traits to BW7 was calculated as carcass percentage (CP), abdominal fat percentage (AFP), breast muscle percentage (BMP), and skin percentage (SP). Subcutaneous fat mainly determines the weight of skin. Therefore, in the present study, SW and SP were considered as indicators of subcutaneous fat weight and percentage. Intramuscular fat content of part of the breast muscle, pectoralis minor, was measured by means of extraction in a Soxhlet apparatus with petroleum ether (AOAC, 1990), and intramuscular fat percentage (IFP) was calculated. Because of experimental limitations, IFP was measured on 1,467 birds.
Genetic Analyses Descriptive statistics, including the test of the normality of the distribution of traits, were obtained from the univariate procedure of SAS software (SAS institute, 1999). An animal model was used to estimate the genetic parameters of carcass-related traits. In order to find the best model, a likelihood ratio test was used. Based on likelihood ratio test, no difference was found between models with and without maternal and common environmental effects, and interactions among the main effects appeared nonsignificant; therefore, maternal, common environmental effects and interactions were ignored in the final model:

where Yijkm = the performance of the chicken m, si = fixed effect of sex i (i = 1, female and 2, male), fj = fixed effect of feathering j (j = 1, fast and 2, slow feathering), hk = fixed effect of week of hatch k (k= 1,2...13), am = random direct genetic effect of chicken m, and eijkm = random residual effect.
The same model was used for all the traits under study. Univariate analyses were used to estimate heritabilities. Multivariate analyses were used to estimate genetic and phenotypic correlations between all combinations of traits. Parameter estimates were obtained using the ASREML software (Gilmour et al., 2000).
RESULTS: Description of Traits
The statistical description of carcass-related traits is summarized in Table 1. Due to missing observations, the number of observations differed among traits. The average of CP, AFP, BMP, SP, and IFP were 66.09, 3.38, 13.03, 1.97, and 1.4%, respectively. The effect of sex was significant for all traits (Table 1). The mean values for all traits were higher in males than in females except for AFW, AFP, and SP. Results showed that males were leaner than females. Feathering had a significant effect only on BW5, BW7, AFW, and AFP. Averages of BW5 and BW7 for slow-feathering birds (1,270 and 1,989g, respectively) were higher than for fastfeathering birds (1,202, and 1,883g, respectively). On the other hand, averages ofAFWand AFP for slow-feathering birds were lower (64.1 g and 3.2%, respectively) than for fast-feathering birds (68.3 g and 3.7%, respectively). These results showed that slow-feathering birds were leaner than fast-feathering birds. The effect of week of hatch was significant for all traits. The mean values for carcass traits were higher in birds with older mothers than those with younger mothers.
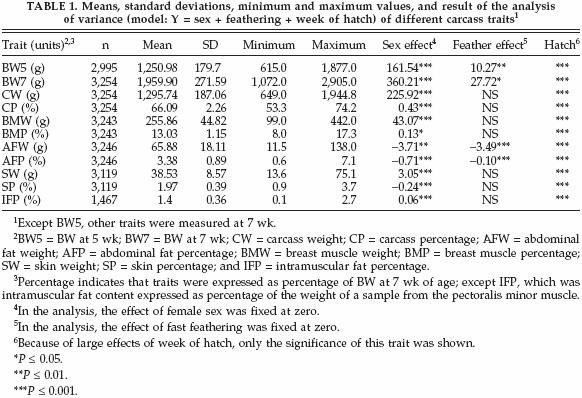
Genetic Parameters
The genetic parameters for carcass-related traits are presented in Table 2. Heritability estimates for AFP, SP, and IFP were 0.71, 0.24, and 0.08, respectively. Heritabilities of other carcass traits were from 0.28 to 0.73. The highest heritabilities (up to 0.73) were obtained for AFW, AFP, and BMP.
In the current study, BW7 showed large genetic correlations with CW and BMW (0.97 and 0.64, respectively), whereas the genetic correlations of BW7 with CP and BMP were smaller (0.22 and 0.12, respectively) (Table 2). Also BW7 showed positive genetic correlations with fat production traits, which were 0.87 for IFP, 0.17 for SP, and 0.13 for AFP. A large genetic correlation between CP and BMP (0.74) was found, and the estimate for CW and BMW was very similar (0.77). The CP and BMP traits had negative genetic correlations with AFP (-0.55 and -0.39, respectively). Genetic correlations among abdominal fat, subcutaneous fat, and intramuscular fat were great between AFW and SW (0.54), whereas the genetic correlation between AFW and IFP was almost zero (0.02).
DISCUSSION
The mean values for all traits were higher in males than in females except for AFW, AFP, and SP. Males were leaner than females. Le Bihan-Duval et al. (1998) found the same results and noted that there is no clear explanation for the difference between sexes, but phenomena such as greater competition between males, different nutritional needs, and greater impact of hormones for fatness in females could be involved. The mean values of carcass-related traits showed that slow-feathering birds were leaner than fast-feathering birds. Slow-feathering birds probably use more energy for stabilizing the body temperature, because of low feather density; therefore their fat deposition is less than fast-feathering birds. In current study, the mean values of all traits were higher in birds with older mothers than those had younger mothers. Peebles et al. (1999) described that older hens lay larger eggs that hatch into larger chickens, and egg weight and hatching weight of chickens are correlated with market age weight.
In the current experiment, the variance in BW5 and BW7 was greater than in commercial situations, which was probably due to the population being a cross between 2 extreme lines with no subsequent selection. According to the review of Chambers (1990), the existence of maternal or dominance effects for BW could be expected, and Chapuis et al. (1996) estimated the size of maternal effects between 2 and 8% of total variability. Clement et al. (2001) demonstrated that if maternal genetic effects exist but are neglected in the model, the direct heritability is overestimated.
In the present study, the number of dams per sire and the total number of observations were limited. This could explain why the maternal effect was not significant. The current estimates of the heritability of BW, CW, and CP were close to previously published estimates for broiler chickens (Le Bihan-Duval et al., 1998, 2001; Chambers, 1990). High heritabilities were found in present study for AFW and AFP. These estimates were in agreement with estimates reported by Leenstra and Pit (1988), Griffin (1996), Le Bihan-Duval et al. (1998), and Rance et al. (2002) and ranged from 0.45 to 0.85.
In the current study, positive genetic correlations were observed between BW and fat production traits (AFW, SW, and IFP). Almost all estimates of the genetic correlations of BW with AFW and AFP that have been published are unfavorably positive (Chambers, 1990; Le Bihan-Duval et al., 1998; Deeb and Lamont, 2002). Sinsigalli et al. (1987) noted that abdominal and subcutaneous fat deposition in chickens selected for rapid growth is associated with changing concentrations of hormones and neural control mechanisms (hunger-satiety control mechanisms) that regulate feed intake. Therefore, most modern meattype chickens eat more than they require for muscle growth and maintenance. This excessive energy intake leads to increasing fat deposition in the body.
The large differences between genetic and phenotypic correlations for carcass traits may imply a relatively large influence of environmental conditions for these traits. In present study, AFW and SW were highly correlated, but the genetic correlation between AFW and IFP was almost zero. Evans (1977) estimated that abdomen and skin store the major part of body fat in poultry and that they are highly correlated. On the other hand, Cahaner et al. (1986) found that considerable changes in the size of adipose tissue are not accompanied by substantial changes in inter- or intramuscular fat in the chicken. Hrdinka et al. (1996) described that the abdominal and subcutaneous fat had very similar fatty acid patterns and differed significantly from the composition of the fat extracted from breast.
These results, together with the results of current study, indicated that selection for reducing AFW does not automatically result in a change in IFP and probably meat quality. The IFP trait showed positive genetic correlations with BW, CW, CP, BMW, and BMP. Selection for increased BMW or BW, therefore, will result in increased IFP. Marshall (1994) reviewed the literature and suggested that selection for beef tenderness would be compatible with selection for improvement in most other carcass traits. This conclusion was supported by Wulf et al. (1996), who reported positive genetic relationships among production, carcass traits, and beef palatability traits.
The results of the present study showed that the genetic correlation between BW and BMP was 0.12. This finding was in agreement with Le Bihan-Duval et al. (1998), who reported low genetic correlations between BW and BMP (0.15 to 0.2).
Therefore, including BMP in the selection index could be justified, especially for lines that are used for further processing. However selection for BMP not only increases breast muscle production but also decreases AFW and AFP, because there is a negative genetic correlation between this trait and fat production traits. Ricard and Tourille (1988) reported the negative genetic correlations between these traits. Therefore, BMP could be an economically important trait in these lines.
In conclusion carcass composition could be improved by selection for increasing CP and BMP and decreased AFW and AFP without decreased IFP. But these kinds of selections in practice have been accompanied by a number of negative consequences, including increased incidence of leg problems and ascites (Julian, 1998). These effects are likely related to disruption of physiological homeostasis. However, more investigations are needed for clarification of physiological relationship between fat storage in different parts of carcass (e.g., abdominal fat) and animal health.
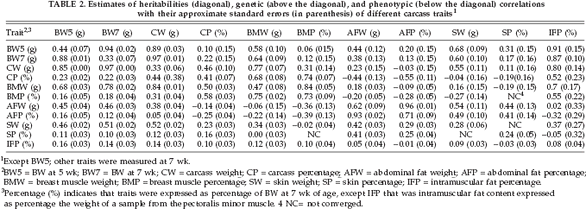
ACKNOWLEDGMENTS
The authors thank Nutreco breeding Research Center for data collection; Bart Ducro, Abe Huisman, and Abbas Pakdel (Wageningen University) for their comments and suggestions; and Islamic Republic of Iran, Ministry of Science, Research, and Technology (MSRT) for financial support.
REFERENCES
Aho, P. 2001. Subject: Poultry Elite. http://www.wattnet.com/ Archives/Docs/501wp20.pdf. Watt Poultry USA. Accessed May 2001.
AOAC, 1990. Official Methods of Analysis: Fat or Ether Extract in Meat. 15th ed. Association of Official Analytical Chemists, Washington DC.
Cahaner, A., Z.Nitsan, and I. Nir. 1986. Weight and fat content of adipose content of adipose and nonadipose tissues in broilers selected for or against abdominal adipose tissue. Poult. Sci. 65:215–222.
Chambers, J. R. 1990. Genetics of growth and meat production in chickens. Pages 599–643 in Quantitative Genetics and Selection. R. D. Crawford, ed. Poultry Breeding and Genetics. Elsevier, Amsterdam.
Chapuis, H.,M. Tixier-Boichard, Y. Delabrosse, and V. Ducrocq. 1996. Multivariate restricted maximum likelihood estimation of genetic parameters for production traits in 3 selected turkey strains. Genet. Sel. Evol. 28:197–215.
Chizzolini, R. E. Zanardi, V. Dorigoni, and S. Ghidini. 1999. Calorific value and cholestrol content of normal and low fat meat and meat products. Trends Food Sci. Technol. 10:119–128.
Clement, V., B. Bibe, E. Verrier, J. M. Elsen, E. Manfredi, J. Bouix, and E. Hanocq. 2001. Simulation analysis to test the influence of model adequacy and data structure on the stimation of genetic parameters for traits with direct and maternal effects. Genet. Sel. Evol. 33:369–395.
Deeb, N., and S. J. Lamont. 2002. Genetic architecture of growth and body composition in unique chicken populations. Heredity 93:107–118.
Evans, A. J. 1977. The growth of fat. Pages 29-64 in Growth and Poultry Meat Production. K. N. Boorman and B. J. Wilson, ed. British Poultry Science, Edinburgh.
Ewart, J. 1993. Evaluation of genetic selection techniques and their application in the next decade. Br. Poult. Sci. 34:3–10.
Fotsa, J. C., P. Merat, and A. Bordas. 2001. Effect of the slow (K) or rapid (k+) feathering gene on body and feather growth and fatness according to ambient temperature in a Leghorn × brown egg type cross. Genet. Sel. Evol. 33:659–670.
Gilmour, A. R., R. Thompson, B. R. Cullins, and S. J. Welham. 2000. ASREML Reference Manual. NSW Agriculture, Orange, Australia.
Griffin, H. D. 1996. Understanding genetic variation in fatness in chickens. Annual report. Roslin Institute, Edinburgh.
Griffin, H. D., K. Guo, D. Windsor, and C. Goddard. 1991. Why are young broiler chickens fatter than layer-strain chicks? Comp. Biochem. Physiol. 100A:205–210.
Havenstein, G. B., P. R. Ferket, and M. A. Qureshi. 2003. Carcass composition and yield of 1957 versus 2001 broilers when fed representative 1957 and 2001 broiler diets. Poult. Sci. 82:1509–1518.
Hrdinka, C.,W. Zollitsch, W. Knaus, and F. Lettner. 1996. Effects of dietary fatty acid pattern on melting point and composition of adipose tissues and intramuscular fat of broiler carcasses. Poult. Sci. 75:208–215.
Julian. R. J. 1998. Rapid Growth Problems: ascites and skeletal deformities in broilers. Poult. Sci. 77:1773–1780.
Le Bihan-Duval, E., C. Berri, E. Baeza, N. Millet, and C. Beaumont. 2001. Estimation of the genetic parameters of meat characteristics and of their genetic correlations with growth and body composition in an experimental broiler line. Poult. Sci. 80:839–843.
Le Bihan-Duval, E., S. Mignon-Grateau, N. Millet, and C. Beaumont. 1998. Genetic analysis of a selection on increased body weight and breast muscle weight as well as on limited abdominal fat weight. Br. Poult. Sci. 39:346–353.
Le Bihan-Duval, E., N. Millet, and H. Remingnon, 1999. Broiler meat quality: Effect of selection for increased carcass quality and estimates of genetic parameters. Poult. Sci. 78:822–826.
Leenstra, F. R., and R. Pit. 1988. Fat deposition in a broiler sire strain. 3. Heritability of and genetic correlations among body weight, abdominal fat, and feed conversion. Poult. Sci. 67:1–9.
Marshall, D. M. 1994. Breed differences and genetic parameters for body composition traits in beef cattle. J. Anim. Sci. 72:2745–2755.
Nishimura, T., A. Hattori, and K. Takahashi. 1999. Structural changes in intramuscular connective tissue during the fattening of Japanese Black cattle: effect of marbling on beef tenderization. J. Anim. Sci. 77:93–104.
Oddy, V. H., G. S. Harper, P. L. Greenwood, and M. B. McDonagh. 2001. Nutritional and developmental effects on the intrinsic properties of muscles as they relate to the eating quality of beef. Aust. J. Exp. Agric. 41:921–942.
Peebles, E. D., S. M. Doyle, T. Pansky, P. D. Gerard, M. A. Latour, C. R. Boyle, and T. W. Smith. 1999. Effects of breeder age and dietary fat on subsequent broiler performance. 1. Growth, mortality, and feed conversion. Poult. Sci. 78:505– 511.
Rance, K. A., G. M. Mcentee, and R. M. Mcdeitt. 2002. Genetic and phenotypic relationships between and within support and demand tissues in a single line of broiler chicken. Br. Poult. Sci. 43:518–527.
Ricard, F. H., and C. Tourille. 1988. Selection for leanness and carcass quality. Pages 377–386 in Leanness in Domestic Birds: Genetic Metabolic and Hormonal Aspects. B. Lecelercq and C. C. Whitehead, ed. Butterworths, London.
SAS Institute. 1999. SAS User’s Guide: Statistics, SAS Institute Inc., Cary, NC.
Sinsigalli, N. A., J. P. McMurty, J. A. Cherry, and P. B. Siegel. 1987. Glucose tolerance, plasma insulin an d immunoreactive glucagon in chickens selected for high and low body weight. Nutrition 117:941–947.
Vereijken, A. L. J. 1992. Genetic of body conformation and breast meat yield in broilers. Pages 98–100 in Proceedings of the 19th World’s Poultry Congress. Vol. 3. WPSA, Amsterdam.
Wulf, D. M., J. D. Tatum, R. D. Green, J. B. Morgan, B. L. Golden, and G. C. Smith. 1996. Genetic influences on beef longissimus palatability in Charolais- and Limousin-sired steers and heifers. J. Anim. Sci. 74:2394–2405.
September 2006